Comparison of meso- to microscale coupling
Authors: Clementine Le Guennic, EDF; Bjarke Tobias Olsen, DTU
One of the use cases within the project HPCWE focuses on the simulation of an inland testing site with relatively complex topography: Perdigão. Of the three participants in the benchmark, two perform computational fluid dynamics (CFD) simulations: DTU with the Ellipsys-3D software, and EDF with Code_Saturne.
Atmospheric flow simulations with CFD codes require boundary conditions, which can be provided
- through the use of academic wind and temperature profiles. While these are easy to produce, they are not necessarily representative when studying an orographically complex site;
- through chaining with a mesoscale model such as WRF. This allows for time-varying boundary conditions that can accurately reflect the changing weather patterns.
For this use case, WRF simulations have been produced by DTU and provide the boundary conditions used by both micro-scales codes. However, different strategies can be applied to effectively implement the meso- to microscale coupling. The use case is an opportunity to compare two such strategies, as DTU and EDF rely on different approaches.
A common approach is to directly use WRF output to define the lateral boundary conditions (LBCs) of the CFD domain. The variables of interest (generally the velocity components, temperature and turbulent variables which may vary depending on the turbulence model) are then interpolated in space to be adapted to the finer mesh of the CFD domain (see Figure 1 for a schematic principle).
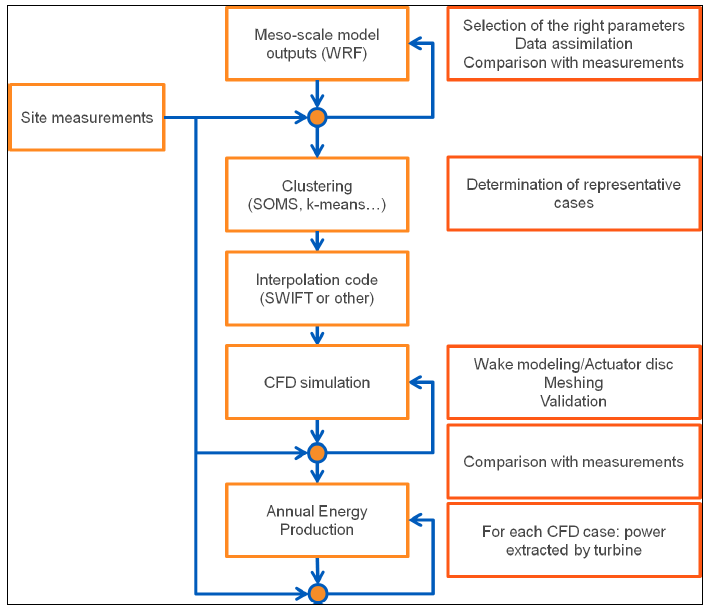
This method is used by EDF within the in-house WRAPP methodology, which has been developed to assess annual energy production in wind farms, and can be decomposed in several steps:
- WRF simulations: ideally over a long time period, since the goal is to be able to reproduce the wind climate;
- clustering: to ensure a reasonable number of CFD simulations, the WRF output is grouped into a predetermined number of classes using a clustering algorithm – k-means for this study. This step must be performed carefully, as both the number of classes and the data extracted from WRF to perform the clustering have been shown to impact the accuracy of the outcome. While previous WRAPP studies ranged from 200 to 500 classes in general, the Perdigão case will allow us to go up to 1500 classes. A future blog post will focus on the challenges of proper clustering. Once the clustering step is done, the best matching unit (BMU) of each class is extracted, and the corresponding WRF profiles are reconstructed.
- microscale simulations: one CFD computation is run for each class obtained at the end of the clustering step. Combined with the respective weight of each class, this allows us to simulate the wind climate.
While directly providing LBCs to the CFD domain has the advantages of being straightforward and lending itself to the simulation of long time-spans through clustering, this method has known challenges. Notably, due to inconsistencies between the meso- and micro-scale domains, the flow in the finer domain requires a spin-up region to adjust; results in this region are generally error prone and unreliable (Warner, 2011). This is particularly important for mountainous areas, where mismatches in ground elevation between WRF and the CFD code can lead to gravity waves reflection.
Another methodology for dynamical coupling is the model coupling via momentum and temperature tendencies. DTU has been working on this approach (Olsen, 2018), in which the fine-scale domain is forced via source terms, rather than LBCs, consisting of relevant momentum and temperature tendencies extracted from the large-scale model domain. This approach was used for Single-Column Model (SCM) evaluation (e.g. Bosveld et al., 2014; Sanz Rodrigo et al., 2017) to investigate turbulence closure models for a diurnal cycle where geostrophic shear and advection, of both momentum and temperature, played a role in forming a nocturnal-LLJ at the Cabauw mast in the Netherlands. To date, the use of coupling with tendencies for non-idealized cases in complex terrain remains fairly limited. The methodology requires the use of periodic boundary conditions, and hence smoothing of topography near the domain boundaries.
Coupling with tendencies has several desirable properties:
- the spin-up, or fetch region of LBCs approaches, is replaced by a smoothed region near the boundary and periodic boundary conditions, resulting in fewer issues related to model inconsistencies and no need for Rayleigh damping at the lateral boundaries;
- concerns related to applying a divergent velocity field to the boundaries are avoided;
- a background pressure gradient is often used to drive CFD models, forcing the flow via body-forces, so this approach also fits well into the existing methodologies.
However, the methodology of extracting tendencies from a mesoscale model and using them to drive another model is a relatively new technique, so it remains a question whether it can be widely generalized.
To obtain long-term wind climates, DTU will run EllipSys3D for a number of carefully selected days, that when combined matches the long-term conditions at the site.
The Perdigão use case presents a good opportunity to:
- compare these two approaches on a single day of measurement;
- compare them and their ability to reproduce the local wind climate over a longer time period;
- and finally, to compare CFD approaches to WRF-LES computations performed by HPCWE project partner Vortex.
The necessary WRF simulations performed by DTU will be presented in detail in a future blog update.
References
Warner, T. T., 2011. “Quality assurance in atmospheric modeling”, Bulletin of the American Meteorological Society, 92(12):1601–1610.
Olsen, B.T., 2018. “Mesoscale to microscale coupling for determining site conditions in complex terrain” (Doctoral dissertation, PhD thesis, DTU Wind Energy, https://doi. org/10.11581/00000036).
Bosveld, F. C., Baas, P., van Meijgaard, E., de Bruijn, E. I. F., Steeneveld, G. J., and Holtslag, A. A. M., 2014. ”The Third GABLS Intercomparison Case for Evaluation Studies of Boundary-Layer Models. Part A: Case Selection and Set-Up”, Boundary-Layer Meteorology, 152(2):133–156.
Sanz Rodrigo, J., Churchfield, M., & Kosovic, B., 2017. “A methodology for the design and testing of atmospheric boundary layer models for wind energy applications”, Wind Energy Science, 2(1), 35–54.